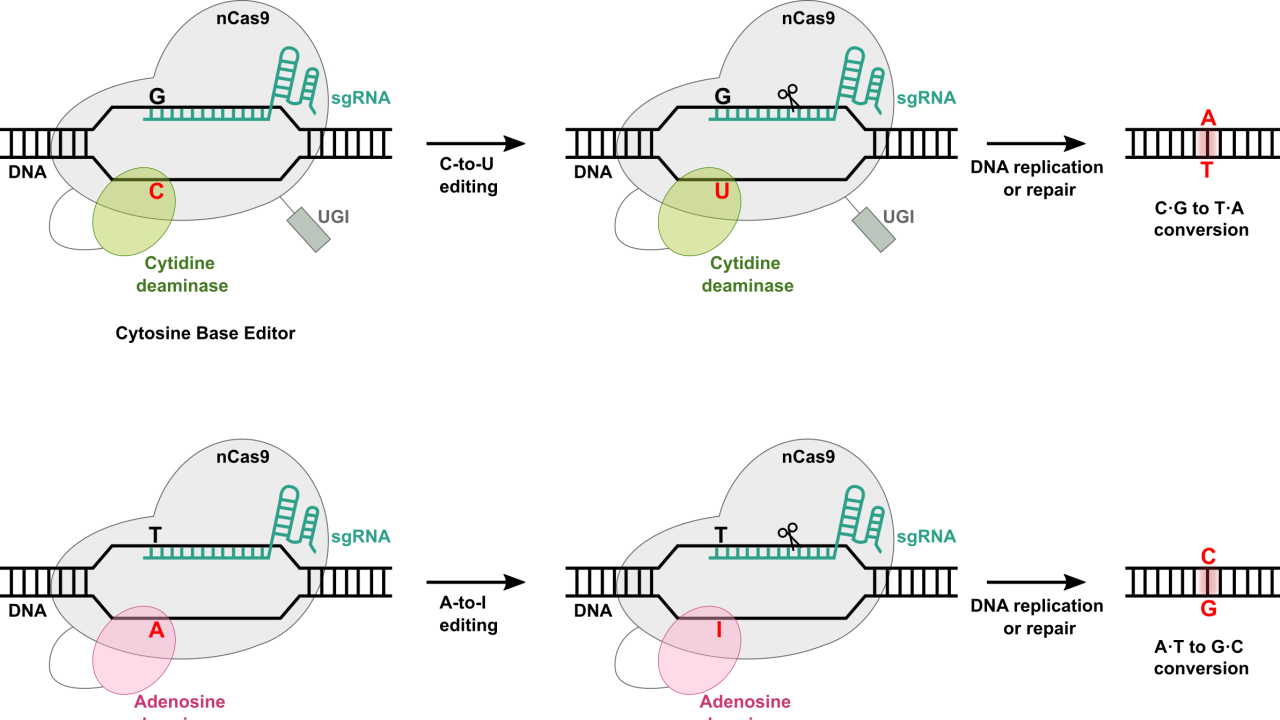
DNA Base Editors for Genome Editing
DNA Base Editors for Genome Editing
by SeHee Park
Genome editing refers to the process that modifies genomic DNA sequence by insertion, deletion, or modification. Several genome editing systems such as TALENs (Transcription activator-like effector nucleases) and ZFNs (Zinc finger nucleases) have been developed and used for genome editing. However, the discovery of CRISPR-Cas9 (Clustered regularly interspaced short palindromic repeats and CRISPR-associated protein 9) system has revolutionized the field of genome editing because it is a faster, easier, and more efficient strategy for genome editing compared to other genome editing systems. The CRISPR-Cas9 system was adapted from the immune system of bacteria as a defense mechanism. It consists of two main components, one of which is a single guide RNA (sgRNA). sgRNA contains both a target-specific sequence that can bind to target DNA and a scaffold sequence that allows for proper interaction with a Cas9 enzyme, which is the other component of CRISPR-Cas9 system. When sgRNA is bound to a target DNA sequence, the Cas9 enzyme can cut two strands of DNA at a specific location, creating what is known as Double-Strand Break (DSB). This DSB is what allows for researchers to modify genomic DNA. However, CRISPR-Cas9 genome editing has low efficiency and DSB can result in unwanted insertions or deletions (indels) within a target DNA which can be problematic (Figure 1).
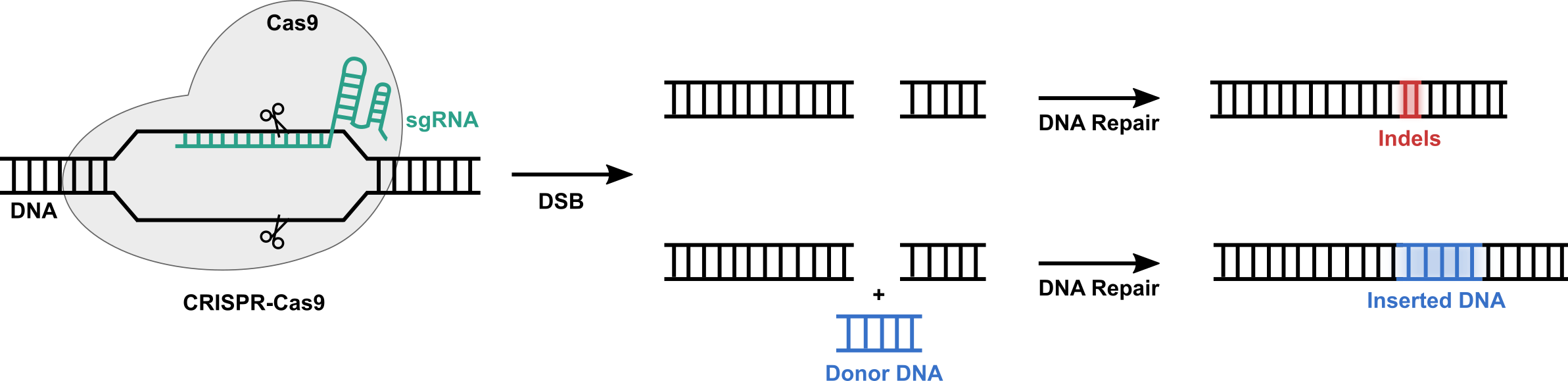
To overcome this issue, other CRISPR-Cas-based genome editing tools have been developed. DNA base editors are genome editing tools based on the CRISPR-Cas system. DNA base editors also use sgRNA and Cas9 protein (or other variants of Cas protein), which are the main components of the CRISPR-Cas system. However, Cas9 protein used for DNA base editors is mutated to what is referred to as nCas9, which cuts only one of the DNA strands instead of both strands. This single strand break mediated by nCas9 reduces indel formation and increases the efficiency of genome editing by base editors. Importantly, for base editors, nCas9 protein is further fused with a deaminase protein to carry out a single base change within DNA. As explained above, sgRNA directs nCas9 protein to the target DNA and this also brings a deaminase protein that is fused to nCas9 protein close to the target DNA so that it can catalyze single base conversion within a target DNA. These base editors are a more efficient strategy for genome editing, especially for a single base change in the genome and creates less indels. There are two types of DNA base editors: Cytosine Base Editor (CBE) and Adenine Base Editor (ABE) (Figure 2).
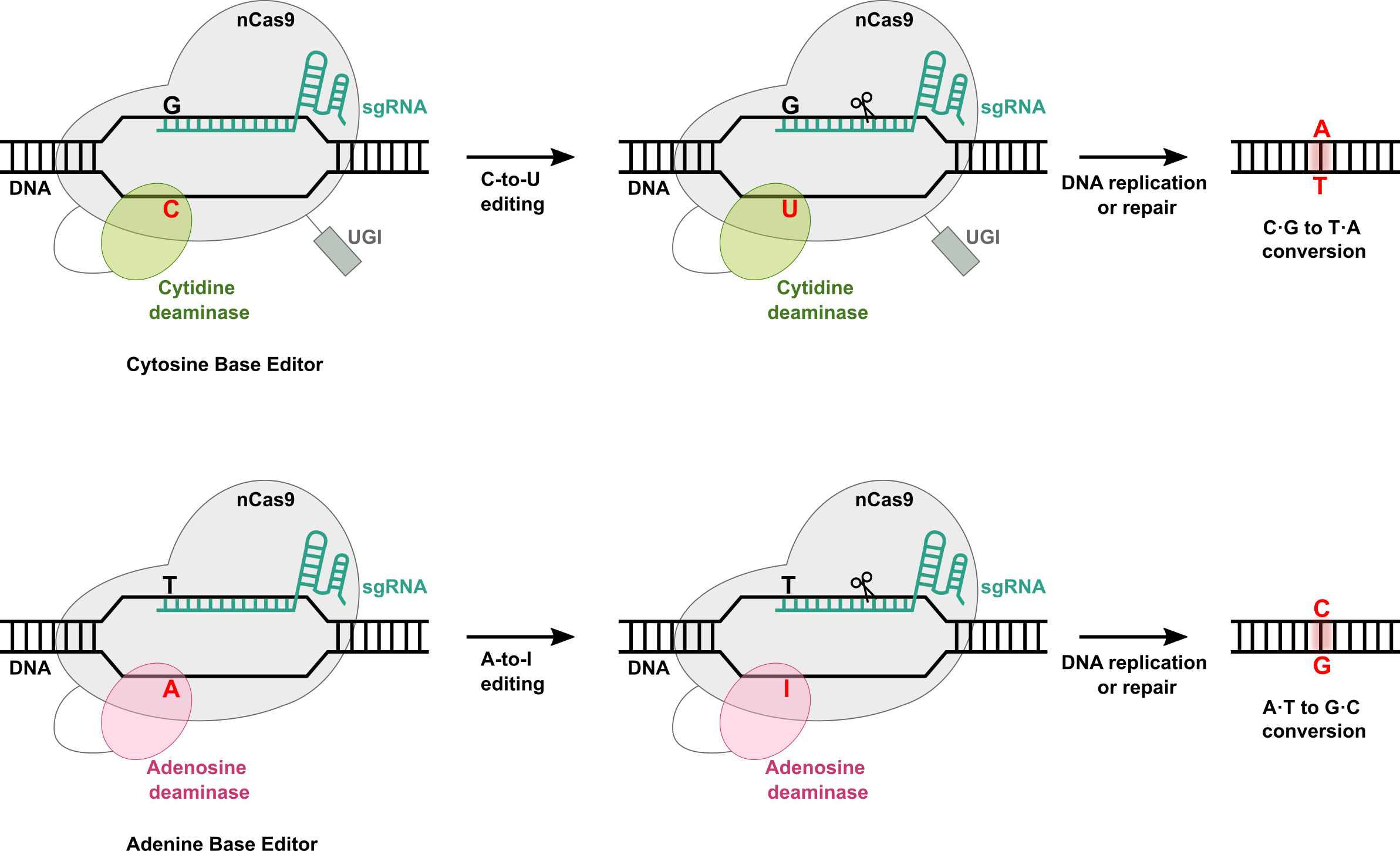
Cytosine Base Editors (CBEs)
Cytosine Base Editor (CBE) coverts Cytosine (C) to Uridine (U) within target DNA. This C-to-U conversion is possible due to the presence of a cytidine deaminase protein that is fused to a Cas protein. CBEs also contain the uracil DNA glycosylase inhibitor (UGI). This is necessary because U is not a typical nucleobase found in DNA but rather in RNA. If there is U in DNA, it is removed by uracil DNA glycosylase (UDG) to prevent mutation in the DNA. Therefore, incorporation of UGI to CBEs can ensure that U is not removed by UDG after conversion of target C-to-U within DNA. Various cytidine deaminases have been used to generate more efficient base modification with less off-target editing and indels. For example, rat APOBEC1 (rAPOBEC1) deaminase was first used for the development of CBE. Later, many other CBEs were developed using different cytidine deaminases such as AID and other APOBEC proteins.
Adenine Base Editors (ABEs)
Adenine Base Editor (ABE) coverts Adenosine (A) to Inosine (I) within target DNA. Inosine is recognized as Guanosine (G) as cellular machinery. Therefore, this results in an A-to-G change within DNA. One challenge of developing ABE was the fact that there is no known deaminase protein that catalyzes A-to-I modification within single-stranded DNA (ssDNA). To overcome this challenge, E. coli TadA (ecTadA), which is a tRNA adenosine deaminase, was chosen for the development of ABE through directed evolution. The resulting evolved ecTadA was fused to nCas9 to generate ABE, which can successfully convert A within ssDNA. Since the development of the first ABE, several different ABEs have been developed to reduce off-target editing and improve editing efficiency.
These DNA base editors along with the CRISPR-Cas system have great potential as therapeutics for genetic diseases especially because many genetic diseases are caused by a single point mutation, which can be readily corrected by base editors. There have been a plethora of studies applying base editors to correct disease-causing mutations in human cell lines. Although there are still several limitations of these base editors including off-target editing in both DNA and RNA and the creation of unwanted indel formations, these studies show the potential of these base editors as therapeutics.
References
Adli, M. The CRISPR Tool Kit for Genome Editing and Beyond. Nat. Commun. 2018, 9 (1), 1911. https://doi.org/10.1038/s41467-018-04252-2.
Anzalone, A. V.; Koblan, L. W.; Liu, D. R. Genome Editing with CRISPR–Cas Nucleases, Base Editors, Transposases and Prime Editors. Nat. Biotechnol. 2020, 38 (7), 824–844. https://doi.org/10.1038/s41587-020-0561-9.
Rees, H. A.; Liu, D. R. Base Editing: Precision Chemistry on the Genome and Transcriptome of Living Cells. Nat. Rev. Genet. 2018, 19 (12), 770–788. https://doi.org/10.1038/s41576-018-0059-1.