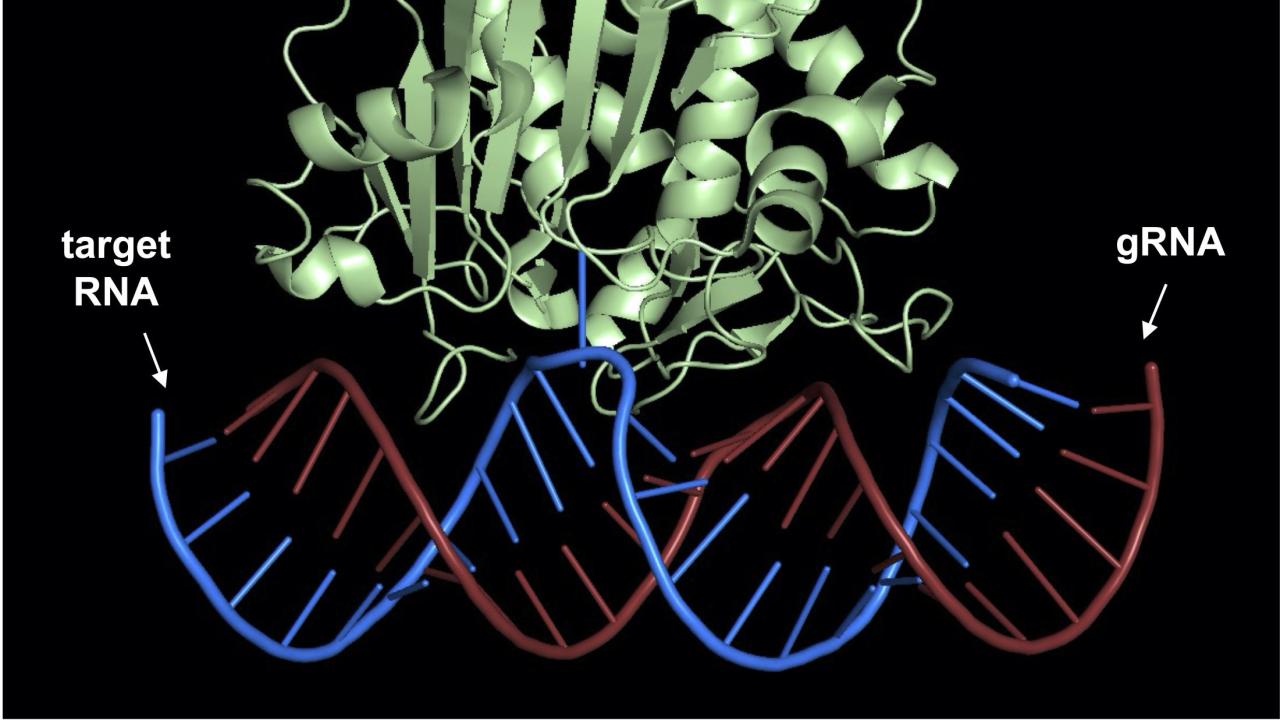
Site-Directed RNA Editing: Unlocking New Avenues for Precision Medicine
In genetics, it is common knowledge that the blueprint of life lies within the intricate structure of DNA. However, a lesser-known but equally important player in the process of gene expression is RNA. From my previous blog, we learned that RNA carries the instructions encoded in DNA and helps to synthesize proteins that dictate the functioning of living organisms. Unlike DNA, which is relatively stable and faithfully replicates itself, RNA is subject to modifications which occur via a process called RNA editing. In this blog post, we will explore RNA editing, its therapeutic applications, advantages and limitations, and the promising implications it holds for biomedical research and the treatment of genetic disorders.
What is RNA editing?
RNA editing is a process that alters the genetic information encoded within RNA molecules (Figure 1). It occurs after transcription, where the RNA transcript is synthesized from DNA, and before translation, where the RNA code is used to build proteins. The most common type of RNA editing involves the conversion of specific bases in nucleotides, which are the building blocks of RNA. This can then result in changes to the genetic code and the protein produced. The two primary types of RNA editing are adenosine-to-inosine (A-to-I) base editing and cytidine-to-uridine (C-to-U) base editing. A-to-I editing involves the deamination of adenosine bases to inosine. This reaction is carried out by enzymes called adenosine deaminases (e.g. ADARs). C-to-U editing, on the other hand, involves the deamination of cytidine bases to uridine by cytidine deaminases (e.g. APOBECs). Both A-to-I and C-to-U conversions lead to changes in the RNA sequence, which can change the meaning of the genetic code and subsequent protein produced.
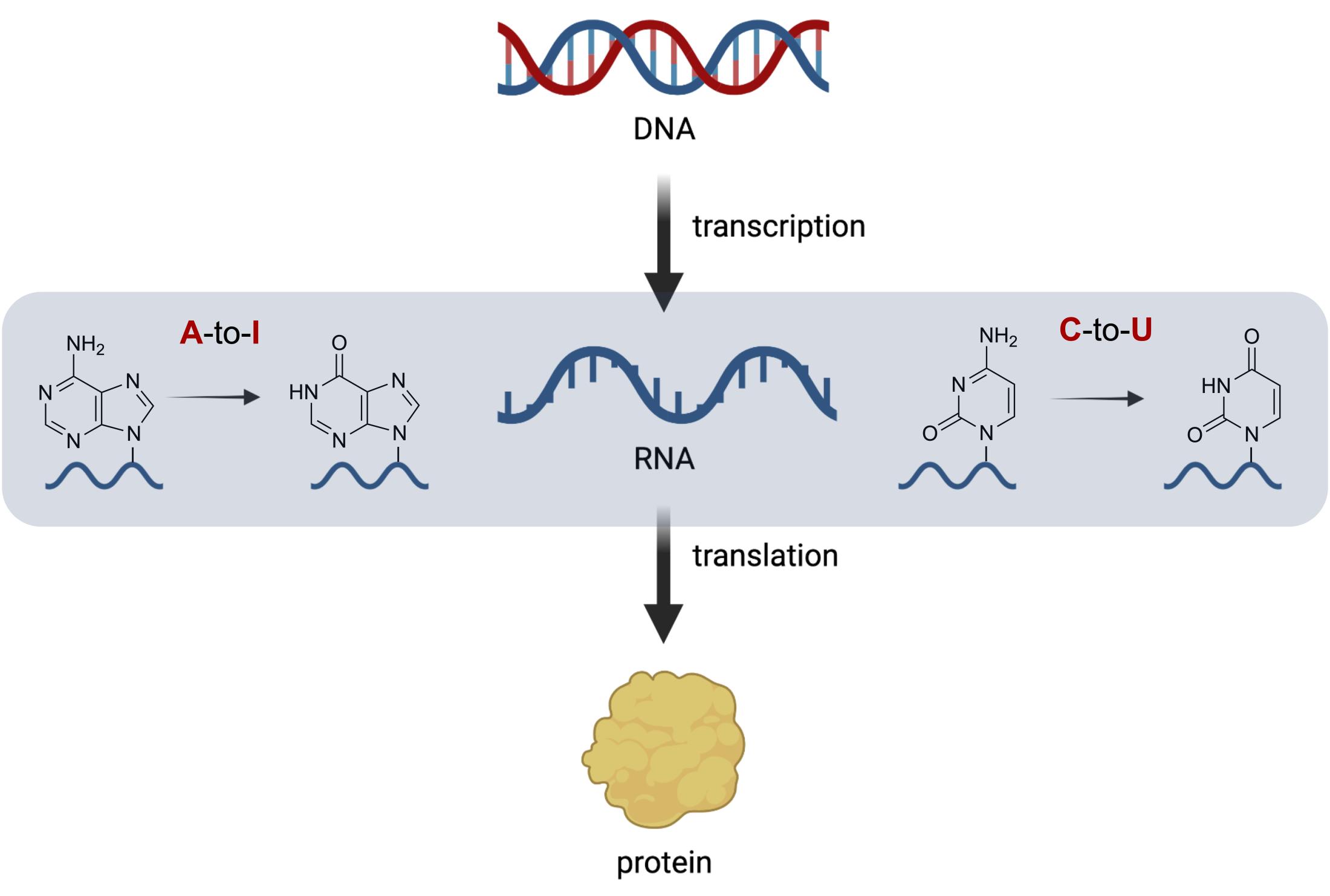
Site-directed RNA Editing (SDRE) as a Therapeutic Approach
The therapeutic potential of RNA editing is particularly exciting. By harnessing this natural process, scientists envision correcting disease-causing genetic mutations in the RNA level to restore normal protein function. This process is called site-directed RNA editing (SDRE). SDRE involves the precise modification of specific nucleotide bases within an RNA molecule to alter the genetic information encoded in the transcript. This approach allows researchers to introduce precise changes in RNA sequences without affecting other regions of the RNA transcript or of the genome, which is the complete set of DNA or genes making up an organism.
The process of SDRE involves several steps (Figure 2). First, the target site within the RNA molecule is identified. This is usually a region where a disease-causing mutation exists or where a specific modification is desired to correct or modify the function of the RNA molecule. Once the target site is determined, the appropriate RNA editing enzyme is selected or engineered to specifically recognize and efficiently edit the desired nucleotide at that site. In some cases, endogenous editing enzymes (already present in the body) are utilized and recruited to the desired site by simply introducing guide RNAs (gRNAs), which are short antisense oligonucleotides (ASOs) complementary to the target RNA (see my previous blog post on RNA-based therapeutics to learn more about ASOs). In other cases, engineered or modified versions of adenosine deaminases or cytidine deaminases with improved selectivity and efficiency, are introduced in the cell together with gRNAs. With the help of the gRNA, the enzyme then recognizes the target site and performs the editing reaction, resulting in the desired modification.

Advantages and Limitations of SDRE
In comparison to DNA whose main function is to store genetic information, RNA is inherently less stable and transient. Hence, one key advantage of RNA editing over DNA or genome editing include safety and reversibility. By selectively editing disease-associated RNA molecules, the focus remains on correcting the genetic defect without permanently changing the genome. Since RNA editing acts directly on RNA molecules, the risk of off-target effects is generally lower compared to genome editing techniques that directly modify the DNA sequence. RNA editing also provides temporal control over gene expression. Unlike genome editing, which permanently modifies the DNA, RNA editing can be dynamically regulated and adjusted as needed. This temporal control enables fine-tuning of gene expression levels and allows for the modulation of protein production at specific times or in specific tissues. Genome editing also typically employs microorganism-derived CRISPR-Cas proteins, which can trigger immune response in the human body compared to the mammalian-derived ADAR and APOBEC enzymes used for RNA editing. These RNA editing enzymes are also relatively small compared to CRISPR-Cas proteins, allowing for easier delivery of RNA editing components compared to genome editing components. This is particularly true in cases where only gRNAs are introduced to recruit RNA editing enzymes that are endogenous to humans.
Despite these advantages, it is important to note that RNA editing also has limitations. The transient nature of RNA molecules requires ongoing administration of editing components for sustained therapeutic effects. Additionally, ensuring precise and efficient delivery of editing tools to target tissues or cells remains a challenge. Off-target effects, although reduced compared to genome editing, still need to be carefully assessed and minimized.
Current State and Future Perspective
Several biotech companies are actively involved in the field of RNA editing, driving innovation and developing technologies for therapeutic applications. A few notable companies engaged in RNA editing research and development are ProQR Therapeutics, Wave Life Sciences, and Shape Therapeutics, which recently formed alliances with Eli Lilly, GSK, and Roche, respectively. These companies are currently conducting preclinical studies using SDRE approaches for the treatment of liver, central nervous system, lung, ocular, and rare diseases. Wave Life Sciences, in particular, is poised to file a clinical trial application to treat alpha-1 antitrypsin deficiency (AATD) using an SDRE approach later this year. The continued progress in RNA editing technologies by these and other companies holds great potential for advancing precision medicine and the development of novel therapeutic interventions for genetic disorders.
Future directions in RNA editing research will involve expanding our understanding of the underlying mechanisms and exploring novel editing techniques. These studies will hold promise for refining the precision and efficiency of SDRE. By improving delivery methods, minimizing off-target effects, and expanding the repertoire of editing enzymes, SDRE has the potential to revolutionize the treatment of genetic disorders and also deepen our understanding of RNA function and regulation.
Note: Images were generated using PowerPoint and BioRender.
References
Diaz Quiroz, J.F.; Siskel, L.D.; Rosenthal, J.J.C. Site-directed AàI editing as a therapeutic tool: moving beyond genetic mutations. RNA. 2023, 29(4):498-505. https://doi.org/10.1261/rna.079518.122
Khosravi, H.M.; Janstch, M.F. Site-directed RNA editing: recent advances and open challenges. RNA Biology. 2021, 18:41-50. https://doi.org/10.1080/15476286.2021.1983288
Matthews, M. M.; Thomas, J. M.; Zheng, Y.; Tran, K.; Phelps, K. J.; Scott, A. I.; Havel, J.; Fisher, A. J.; Beal, P. A. Structures of human ADAR2 bound to dsRNA reveal base-flipping mechanism and
basis for site selectivity. Nature Structural and Molecular Biology. 2016, 23:426−433. https://doi.org/10.1038/nsmb.3203
Sheridan, C. Shoot the messenger: RNA editing is here. Nature Biotechnology. 2023, 41:306-308. https://doi.org/10.1038/s41587-023-01709-8