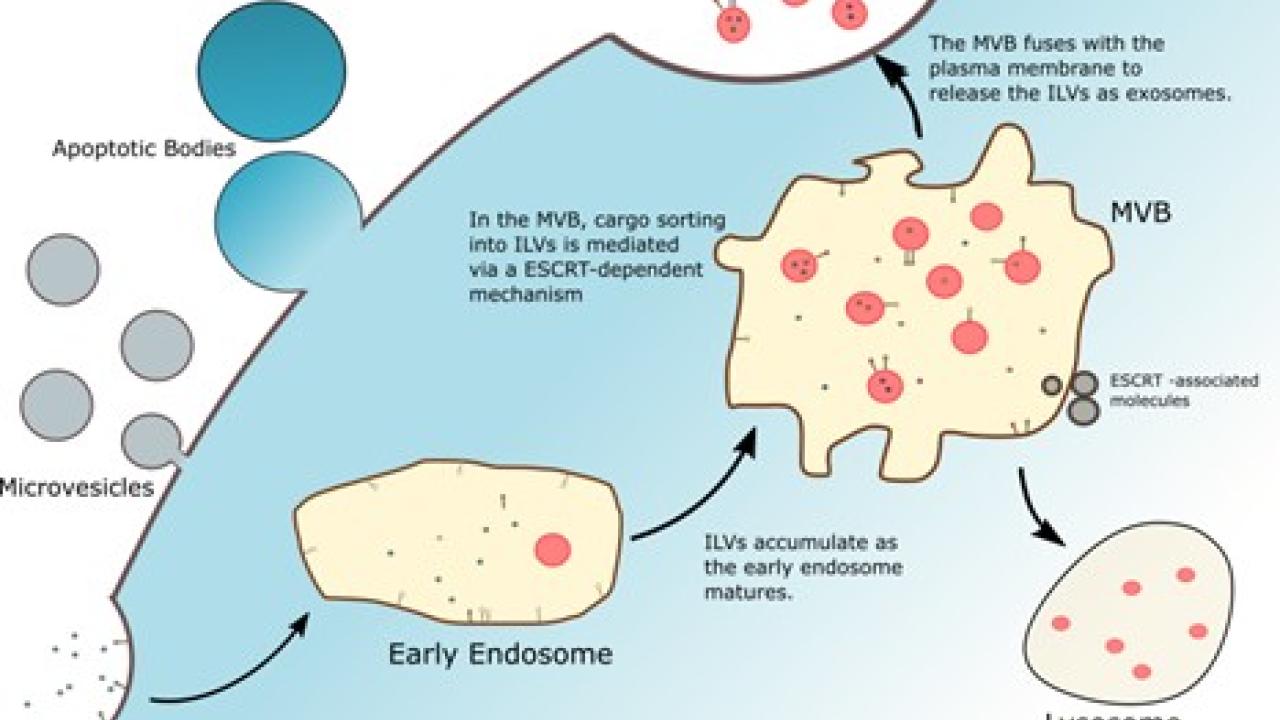
Role of Extracellular Vesicles as the Body’s Messengers
Extracellular vesicles (EVs) are small membranous nanoparticles secreted by all types of cells and can be found in numerous bodily fluids, including blood, urine, cerebrospinal fluid, and more. For years, scientists believed that it functioned more as a waste disposal system for cells, carrying unwanted molecules and metabolites out of the cell and into circulation to be cleared out. But it turns out that one cell’s trash could be another cell’s treasure.
But then in 1996, Raposo et al. demonstrated that these little membranous vesicles secreted by B cells could be used to help T cells proliferate. This landmark paper spurred the advent of a new research field that showed that instead of being biological trash bags, EVs are more the postal service system of our body. They carry bioactive molecules such as proteins and RNA as personalized messages to adjacent cells or even systemically to other organs. Delivery of these messages trigger different biological functions, such as cell growth, immune system modulation, and tissue repair.
Though the EV field is rapidly growing and our understanding of EVs is constantly evolving, there is a general consensus that EVs can be broken down into three subcategories that are differentiated by their biogenesis.
- Apoptotic bodies- Larger vesicles (500 nm- 2 µm) that break off from the cell membranes when cells undergo apoptosis, or programmed cell death
- Microvesicles- Medium sized vesicles (100-100 nm) that pinch off directly from the cell surface,
- Exosomes- Smallest vesicles (50-150 nm) that are derived from multivesicular endosomes (membranous sacs) within the cell
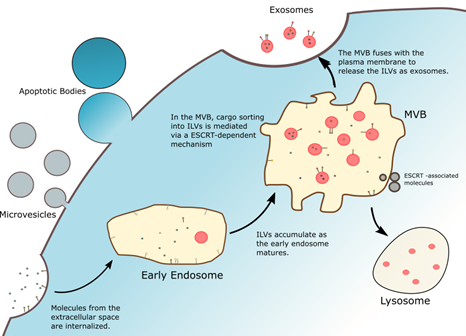
Figure 1. There are three subclasses of extracellular vesicles: microvesicles and apoptotic bodies, which are derived from the plasma membranes of cells, and exosomes, which are derived from endosomal compartments from within the cell.
Role of EVs in Normal Body Homeostasis
So why do EVs matter? Why do we care that EVs can carry messages to other cells?
Our bodies are a delicate balance of competing metabolic processes and biological functions. EVs play an important role in making sure this balance is preserved and does not skew towards one way or the other. In the vascular system, for example, our blood vessels are composed of three layers: the endothelium (the innermost lining made up of endothelial cells), media (large layer of smooth muscle cells that help with vessel contractility), and adventitia (outermost layer which is made of connective tissue that holds the vessel in place). The endothelial cells and smooth muscle cells “talk” to each other via these EVs to make sure that no one layer grows too big in normal, healthy conditions or to stimulate growth if tissue gets injured and needs to be repaired.
EV messaging is a hallmark of normal bodily functions but can also signal warnings for disease prognosis and organ dysfunction. Scientists are now realizing that EVs can be used as diagnostic biomarkers since their vesicle composition and cargo often change to reflect disease pathologies. For example, the onset and progression of cardiovascular diseases can be diagnosed by through the detection of circulating EVs that contain higher abundances of specific microRNAs and proteins. This opens the door for faster and earlier disease diagnosis that could have dramatic implications of patient survival and mortality. In cases of fast-growing cancers, rapid detection using EVs could make all the difference for the patient. Nowadays, use of EV as biomarkers is a popular subfield within EV research. There are now a handful of clinical trials investigating the application of EV biomarkers. For example, a study sponsored by the University of Alabama at Birmingham is studying the correlation between exosomes in blood and urine for Parkinson’s disease. With the emergence of new and more sensitive instrumentation, EVs can be characterized with more detail and new biomarkers can be discovered and correlated with more disease pathologies.
EVs as Natural Therapeutics
Due to their important vital roles in facilitating cell-cell communication and its native presence in the body, it’s no surprise that scientists are looking into whether EVs can be used as a drug. Recent studies show promising results in using EVs for the treatment of cancers, cardiovascular diseases, and immune diseases by attenuating unwanted cell functions. EVs have also been successfully applied to improve tissue recovery following injury or degenerative diseases by promoting cell growth and other regenerative functions to stimulate tissue repair. New engineering strategies have also been used to augment the natural properties of EVs. EVs can be loaded with exogenous drugs to improve therapeutic functionalities or be chemically modified to express specific proteins on its surface to improve its ability to target specific cells. More and more new bioengineering methods are being validated as research in this field continues, with the goal of creating a disease-specific and highly therapeutic EV that is not limited by immune system responses to foreign substances.
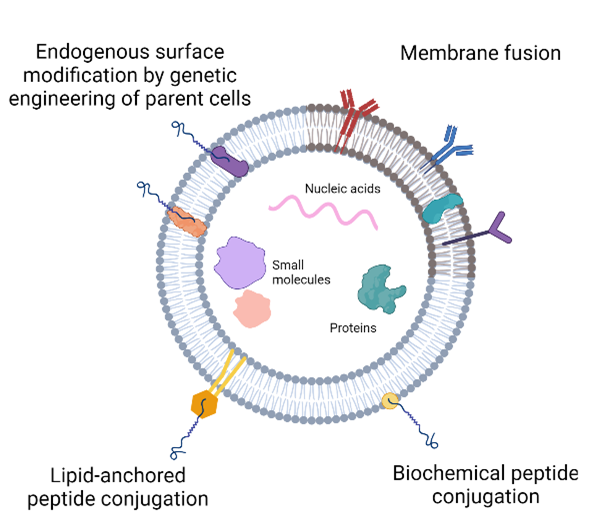
Challenges in EV Research
Though a promising tool for biomedical applications, EV research has met with several challenges that has made effective clinical translation difficult. For one, it can be a very laborious, costly, and time-consuming process to isolate EVs from biofluids. Additionally, the yield of EVs, especially from stem cells, can be extremely low, which makes it difficult to obtain enough EVs for a clinically relevant dose. Additionally, EVs are associated with low heterogeneity and purity. The heterogeneity results from the sensitivity of the origin parent cell that is secreting the EV. Since EVs are released to communicate a cell’s message, the health of the cell makes a huge impact on the composition of the EV. For different batches of EVs to be identical for characterization and standardization purposes, the original batches cells must also be identical. This can be a very difficult logistical issue, especially for large-scale manufacturing, as it can be challenging to keep cells, or any biological entity, in perfectly standardized conditions. Furthermore, the purity of the EVs may be compromised due to the overlapping nature of the EVs with other similarly sized biological substances. Current isolation practices mainly rely on separating EVs from the rest of the biofluids using density or size differences. However, these physical characteristics can overlap with other substances such as lipoproteins, which then results in an EV isolate that is contaminated by other particles as well. Fortunately, scientists are working on strategies to overcome these limitations by designing ways to isolate and purify therapeutically relevant EVs. With constant strides in EV research, we might eventually see EV therapies being administered in clinics soon.
References
Dickhout, A.; Koenen, R.R. Extracellular Vesicles as Biomarkers in Cardiovascular Disease; Chances and Risks. Front. Cardiovasc. Med. 2018, 5, 113, doi:10.3389/fcvm.2018.00113.
Hergenreider, E.; Heydt, S.; Tréguer, K.; Boettger, T.; Horrevoets, A.J.G.; Zeiher, A.M.; Scheffer, M.P.; Frangakis, A.S.; Yin, X.; Mayr, M.; et al. Atheroprotective Communication between Endothelial Cells and Smooth Muscle Cells through MiRNAs. Nat Cell Biol 2012, 14, 249–256, doi:10.1038/ncb2441.
Ramasubramanian, L.; Kumar, P.; Wang, A. Engineering Extracellular Vesicles as Nanotherapeutics for Regenerative Medicine. Biomolecules 2019, 10, 48, doi:10.3390/biom10010048.
Raposo, G.; Nijman, H.W.; Stoorvogel, W.; Liejendekker, R.; Harding, C.V.; Melief, C.J.; Geuze, H.J. B Lymphocytes Secrete Antigen-Presenting Vesicles. J Exp Med 1996, 183, 1161–1172, doi:10.1084/jem.183.3.1161.
West, A.B. LRRK2 and Other Novel Exosome Proteins in Parkinson’s Disease; clinicaltrials.gov, 2018;
Zhang, Y.; Liu, Y.; Liu, H.; Tang, W.H. Exosomes: Biogenesis, Biologic Function and Clinical Potential. Cell Biosci 2019, 9, 19, doi:10.1186/s13578-019-0282-2.