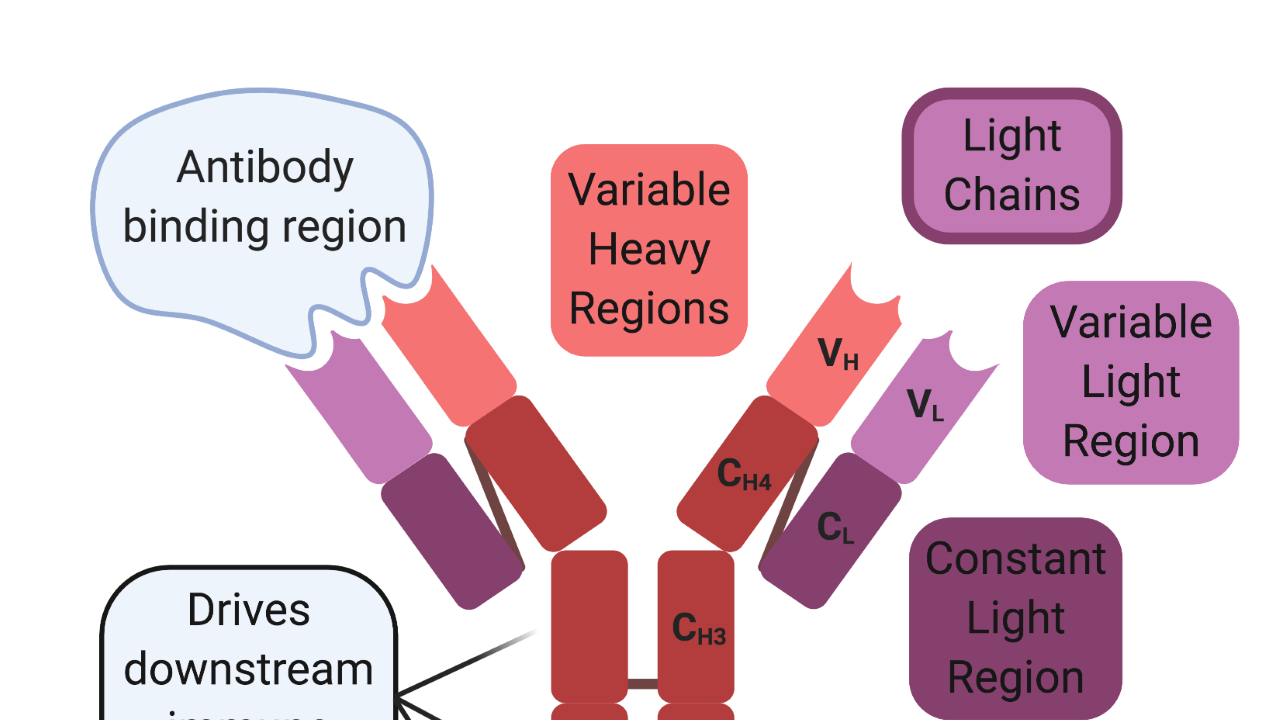
How Do Antibody Therapies Work?
How Do Antibody Therapies Work?
by Jed Bassein
Over the last ~5-10 years, the biomedical field has seen a revolution in biologics through the use of antibody therapeutics. In this blog, I wanted to discuss what antibodies are, how the immune system uses them to combat infections, and how we use them for medical applications and therapeutics.
Antibodies are specialized immunomodulatory proteins also known as immunoglobulins (Ig) that were first discovered in 1890 by Emil von Behring and Shibasaburo Kitasato [1]. They demonstrated that immunity to diphtheria and tetanus could be achieved by injecting animals with serum from previously infected ones. This groundbreaking antitoxin won Behring the Nobel Prize in 1901. Around that same time, Paul Ehrlich (who is considered one of the fathers of immunology) proposed the branched receptor theory for the structure of an antibody. This theory also included the concept for complementary-antigen binding motifs, which is referred to as the “side-chain theory” [2]. Approximately 50 years later, Astrid Fagraeus identified plasma cells as the cellular source for antibodies in 1947 [3]. Ten years after that, Frank Burnet and David Talmage completed a seminal piece of the antibody puzzle by developing the theory of clonal selection. This theory described how somatic clonal mutations could drive antibody selection, affinity, and how aberrant divisions of self-reactive cells could lead to autoimmune disease [4]. Importantly, this theory emphasized the concept of monoclonal antibodies, which states that a single cell was only able to create antibodies for a single target.
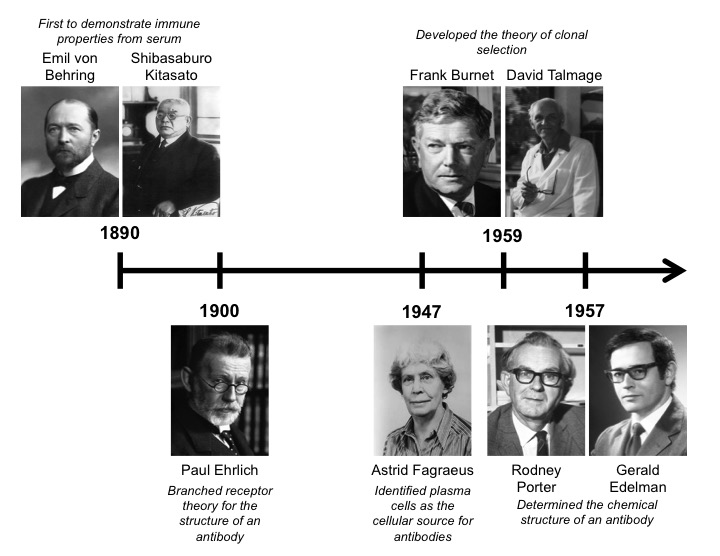
Antibody Structure
In 1959, Rodney Porter and Gerald Edelman independently published the chemical structure of the antibody, which won them the Nobel Prize in 1972 [5,6]. Antibodies are Y-shaped proteins that consist of two antigen-binding heads and one immunomodulatory tail. They are composed of two identical heavy and light chains. Both chains contain constant regions, which are used for intermolecular interactions between the chains, and variable regions, which mediate antibody-binding interactions. Cysteine disulfide bonds hold the two heavy chains together at the constant region to form the immunomodulatory tail. In contrast, the antigen-binding heads are formed between the light and heavy chains. The variable regions of both chains are located at the ends of the beta-helical sheets and mediate antigen-binding interactions, while cysteine disulfide bonds link the constant the region of the light chain to another constant region located near the head of the heavy chain.
The immunomodulatory tail drives downstream immune responses and has evolved in a species-specific manner. Meaning that the constant regions for each mammalian species are distinct from one another. Because of these species-specific differences, antibodies from foreign species can activate an immune response against themselves instead of the intended target. Since most modern antibody therapies begin their lives in preclinical animal models, the intended human antibody must undergo a process of “humanization” to overcome the foreign species antigenic hurdle. “Humanization” is the process of modifying and engineering the therapeutic to be accepted by the human immune system and initiate the desired downstream effector response.
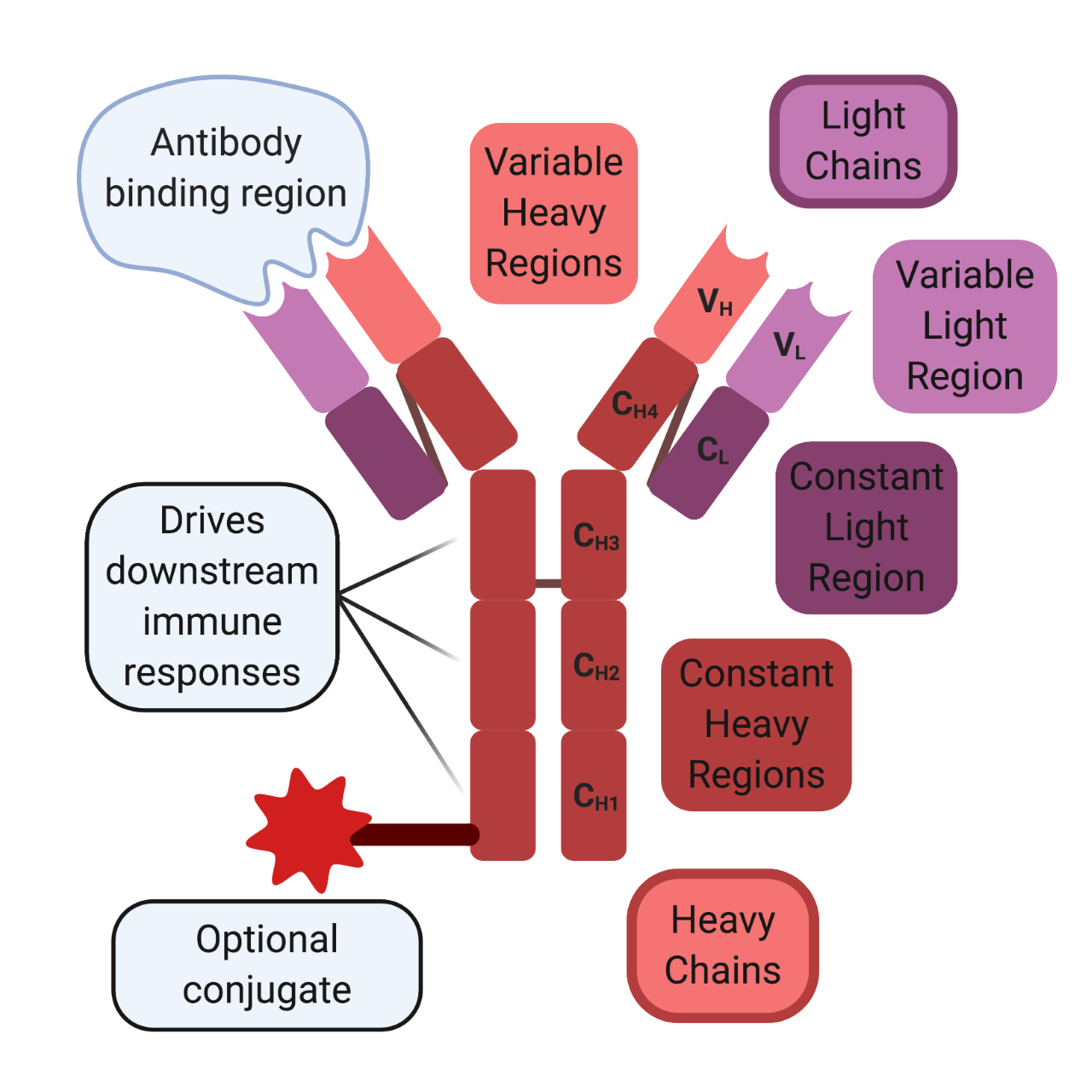
Created with BioRender.com
Antibody Functions
Antibody effector functions fall into 5 main categories, which have been delineated by the general structure of their immunomodulatory heavy chain structures. These antibody isoforms are called IgA, IgD, IgE, IgG, and IgM. Presently, there are several sub-classifications for some of the aforementioned isotypes, but I will not discuss them here. IgD is not secreted and remains bound to the B cell. IgM is usually the first secreted antibody in an immune response and exhibits weak binding to more general pathogen-associated motifs. IgA is the most abundant isoform and is located specifically at mucosal sites. IgG is located in the bloodstream and can cross the placenta to provide fetal immune protection. IgE is effective against parasitic infections but has been implicated in allergic diseases due to the high affinity it exhibits towards its receptor, which is expressed on mast cells and eosinophils.
The applications for antibody-mediated biotechnologies are endless because of their single-target specificity and flexible constant regions. The variable regions can be engineered to target almost anything, while the constant region can be modified to drive downstream effects or be conjugated to a variety of probes, drugs, and other motifs depending on the application. Antibodies are critical for common biological assays, such as flow cytometry, electron microscopy, immunohistochemistry, ELISA, Western blotting, and chromatin immunoprecipitation. In terms of medical applications, antibodies have also had a huge impact and are used in a variety of strategies. They can be used to deliver cytotoxic payloads for cancer therapy, sequester aberrant proteins that drive disorders, modulate cellular frequencies for specific targets, and can provide passive immunity to certain diseases and infections.
Antibody Therapeutics
Many of the current uses for antibody therapeutics were pioneered by immunotherapies for cancer treatment. However, with the global rise in SARS-COV-2 infections, antibody therapeutics have taken the forefront for therapeutic applications. Tocilizumab (Actemra) is a monoclonal antibody against IL-6 that is currently used as a rheumatoid arthritis treatment. It had seen early success for treating severe COVID-19 related pneumonia but was unfortunately unable to meet its primary and secondary endpoints in a Phase III trial called COVACTA by Roche’s subsidiary company, Genentech [7].
More recently, the NIH announced the start of a new Phase III trial called Accelerating COVID-19 Therapeutic Interventions and Vaccines (ACTIV-3) to test a new antibody called LY-CoV555. LY-CoV555 is a monoclonal antibody that was detected in the blood of a patient who recovered from a SARS-COV-2 infection. AbCellera Biologics in Vancouver, British Colombia, identified the antibody in collaboration with the National Institute of Allergy and Infectious Diseases (NIAID) Vaccine Research Center. The trail will begin by testing 300 volunteers who have been hospitalized with mild to moderate COVID-19 in a randomly assigned study testing placebo versus an intravenous (IV) administration of LY-CoV555. If these initial tests prove safe and effective, then the study will be expanded to enroll another 700 volunteers [8].
In summary, antibodies have proven to be powerful, versatile tools of the immune system that can function as an effective therapy for a wide variety of diseases. Antibodies will likely continue to play a major role as we move forward to the future and advance our medical technologies.
References
- Behring, E.v. Ueber das zustandekommen der diphtherie-immunität und der tetanus-immunität bei thieren. 1890.
- Davies, D.R.; Chacko, S. Antibody structure. Accounts of Chemical Research 1993, 26, 421-427.
- Fagraeus, A. Plasma cellular reaction and its relation to the formation of antibodies in vitro. Nature 1947, 159, 499-499.
- Sir frank macfarlane burnet 1899–1985. Nature Immunology 2007, 8, 1009-1009.
- Porter, R.R. Structural studies of immunoglobulins. Science 1973, 180, 713-716.
- Edelman, G.M. Antibody structure and molecular immunology. Science 1973, 180, 830-840.
- Genentech provides an update on the Phase III Covacta trial of Actemra in hospitalized patients with severe COVID-19 associated pneumonia. July 29, 2020.
- NIH launches clinical trial to test antibody treatment in hospitalized COVID-19 patients. August 4, 2020.