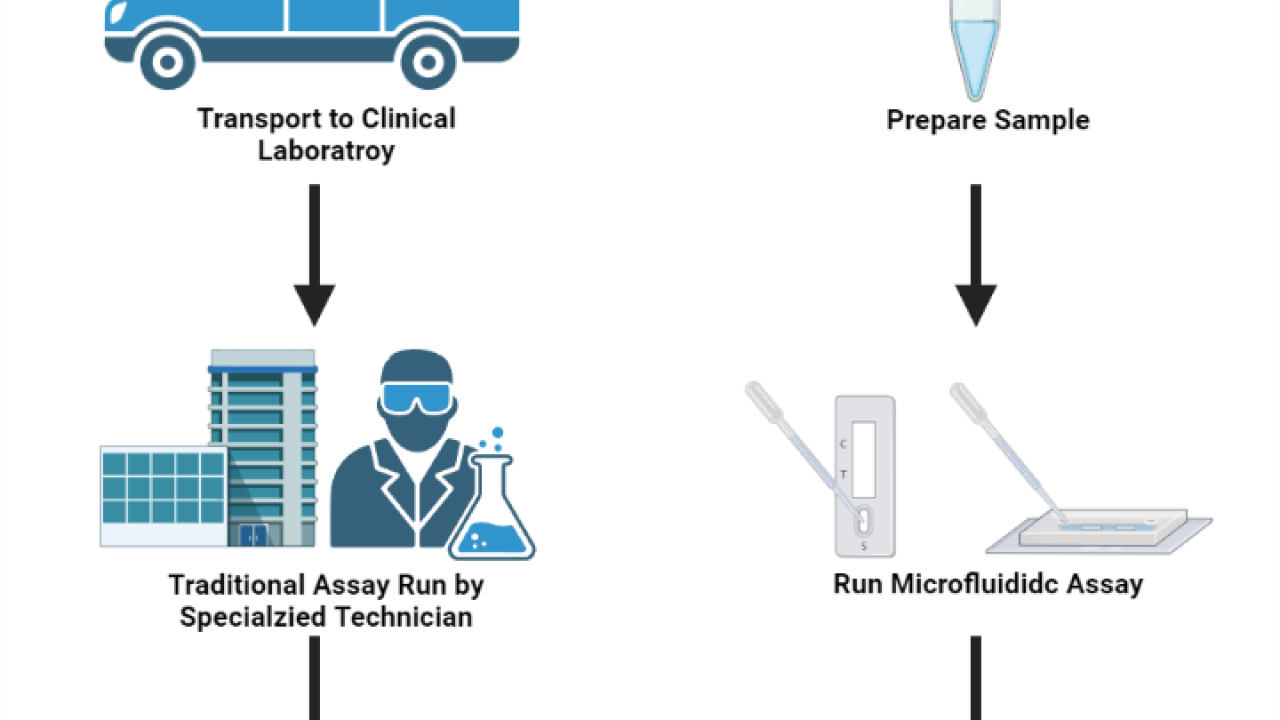
Microfluidics: Doing More with Less - Part 2
Low Resource and Point-of-Care Applications
This is Part 2 of a 3-part series on biological applications of microfluidic devices. Part 1 covered the history, physics, and popular fabrication methods of microfluidic devices. This part will cover the application of microfluidic devices in low resource and point-of-care applications, while Part 3 will discuss the role of microfluidic devices in cutting edge technologies.
In medicine, point-of-care diagnostics refers to the ability to perform complex diagnostic testing procedures at the patient's bedside or clinic in a short period of time. This is in contrast to conventional testing procedures, in which samples are collected at the point of care and then transported to a separate testing facility. Point-of-care diagnostic systems have significant advantages over traditional methods, including a quicker turnaround time and the ability to perform complex tests in low resource settings where access to clinical laboratory settings are unavailable. As we have experienced with the COVID-19 pandemic, the ability to perform rapid on-site detection is of utmost importance for the control of highly infectious diseases. Microfluidic devices have a natural application in these settings due to their low cost, reduced reagent consumption, and low fluidic volumes that can increase diagnostic sensitivity and reduce incubation times. Furthermore, microfluidic devices can be designed in such a way to incorporate multiple steps onto a single device (often referred to as lab-on-a-chip devices) eliminating the need for trained technicians and complex equipment. These lab-on-a-chip devices allow clinicians to rapidly perform complex diagnostic tests in low resource settings where access to power and refrigeration may be limited.
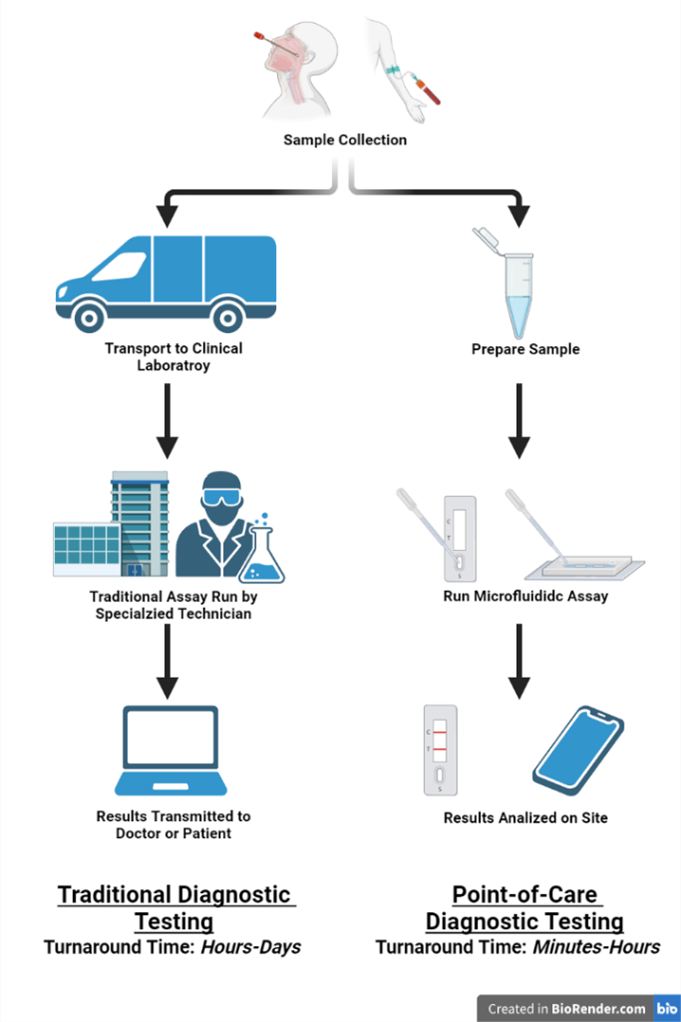
Figure 1. Point-of-care microfluidic diagnostic devices allow for rapid diagnostic testing without the need for sample transportation and specialized equipment and technicians. This is especially beneficial in low resource settings where access to clinical laboratories are limited or unavailable. Created with BioRender.com.
Overview
The detection methods of most point-of-care microfluidic devices are identical to their traditional macroscale counterparts and are largely determined by the target of interest. As microfluidic devices can be optically transparent and integration of electrical components is straightforward, nearly all optical and electrochemical readout methods are compatible. Optical methods are largely based on sandwich ELISAs (enzyme-linked immunosorbent assay), in which antibodies specific to the target of interest (capture antibodies) are fixed to a substrate. In order to perform the diagnostic test, the sample is then added over the substrate, and if the target of interest is present in the sample, it will bind to the capture antibodies. A second antibody specific to the target of interest that is conjugated to an enzyme, or a fluorescent label (detection antibody) is then added and can bind to the target. Finally, a substrate is added, which reacts with the enzyme to produce a color change, and that change in color can be quantified to determine the concentration of the target of interest in the sample (colorimetric or chemiluminescence assay). Alternatively, for fluorescently labeled detection antibodies, the fluorescence signal of the assay can be quantified (fluorescence assay). Another optical method uses aptamers, which are specifically designed single stranded oligonucleotides, instead of antibodies to bind to the target of interest. Upon binding to the target of interest, the aptamer undergoes a conformational shift that significantly alters the fluorescence of a conjugated dye. Electrochemical read out methods also take advantage of specific target binding of antibodies or aptamers, however instead of producing a change in color or fluorescence upon target binding, a change in the electrical properties of the system is produced.
The primary goal of the microfluidic portion of point-of-care devices is to automate the sample preparation and handling to eliminate the need for specialized equipment and highly trained technicians to perform the diagnostic assay. For example, for standard plate-based ELISA assays, a trained technician needs to accurately manipulate microliter volumes of costly and temperature sensitive reagents over the course of a few hours. Using a microfluidic device, the complexity of these steps can be reduced eliminating the need for specialized technicians or equipment. Additionally, the small volume within the microfluidic device significantly reduces the incubation times of the assays, as the diffusion distance between the target of interest and fixed antibody/aptamer is reduced. Combined with the improved cameras and increased processing power of modern smartphones, many of these simple point-of-care microfluidic devices can be run in low resource settings with limited access to refrigeration and electricity. Alternatively, more complex devices can be designed to fully automate the entire assay using multiple pumps and valves to control and direct flow. These devices are beneficial as they eliminate the need for skilled technicians and clinical laboratories thereby reducing the turnaround time, however as these designs typically require specialized equipment, they are not suitable for low resource settings.
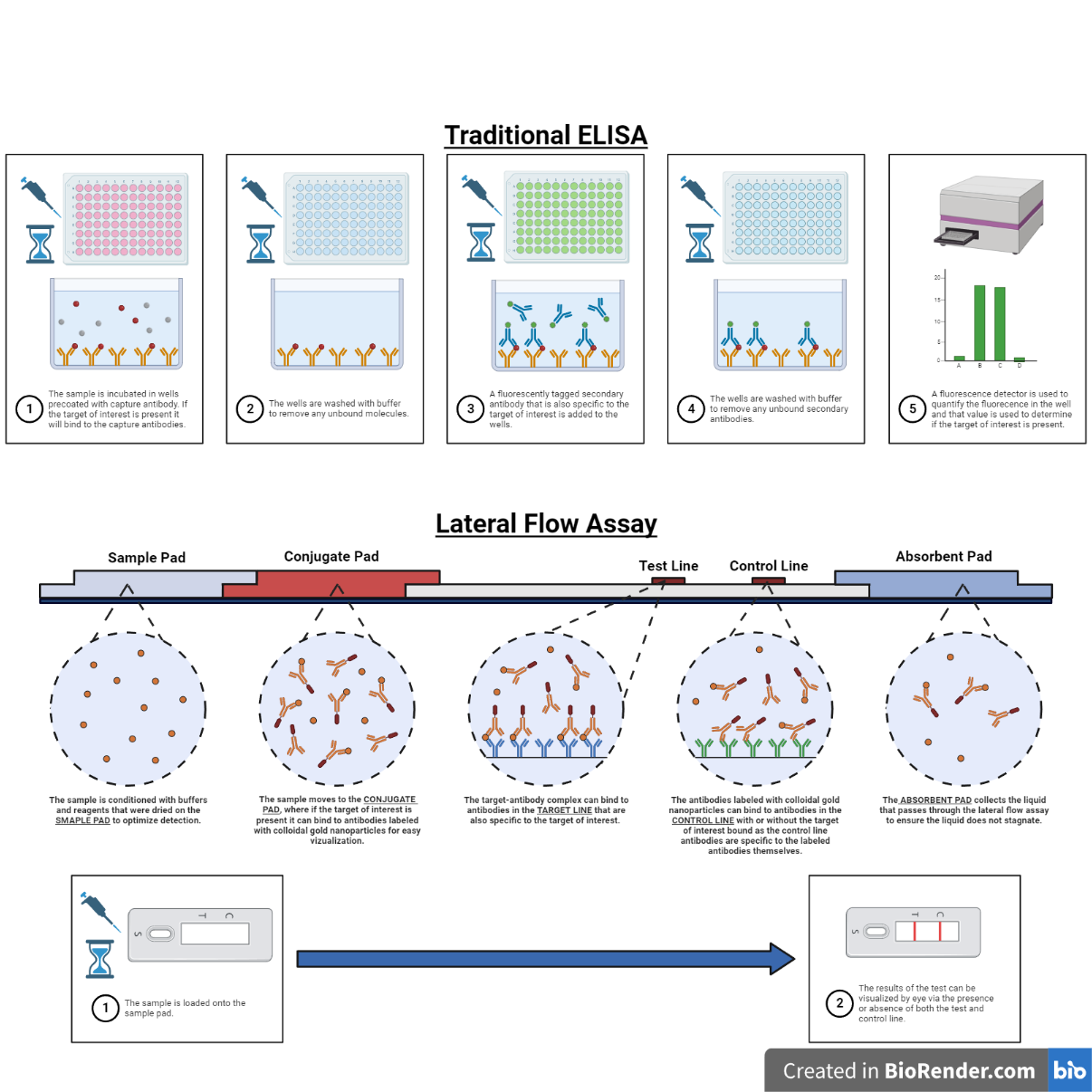
Figure 2. Comparing the workflow and underlying principles for traditional ELISA detection assays and point-of-care lateral flow assays. Created with BioRender.com.
Paper-Based Microfluidics
Paper-based microfluidics is a popular alternative to microchannel based microfluidics in point-of-care devices. This is especially true for point-of-care devices designed for use in low resource settings or at home use. Instead of small volumes of liquid flowing through micrometer scale channels, the liquid is instead wicked through defined areas of cellulose or nitrocellulose paper. In point-of-care applications, paper-based microfluidic devices have significant advantages over traditional microfluidic devices that largely overshadow the loss of precise fluidic control. These include reduced cost, the ability to “print” and dry reagents at specific locations, and the wicking action of the paper that eliminates the need for complex pumps to induce flow. The simplest version of these devices are called lateral flow assays and are the basis for many common at home diagnostic kits including pregnancy and rapid COVID antigen tests.
The detection methods of lateral flow assays are the same as macroscale and channel based microfluidic assays. ELISA based lateral flow assays consist of five regions connected by a strip of paper. An absorbent pad sits on one side of the assay that collects the liquid once it has wicked through the assay and prevents backflow or stagnation of the sample liquid. On the other end of the assay is the sample pad where the liquid sample is loaded. In addition to holding the sample, the sample pad often contains other dried reagents such as buffers or detergents that dissolve back into once the sample is added that optimize the detection capabilities of the assay. Connected to the sample pad is the conjugate pad, which contains the labeled antibody specific to the target of interest. The antibodies are dried within the conjugate pad along with other stabilizers and buffering reagents that help the antibodies maintain their native conformation when dried and help re-solubilize them upon wetting. The size and thickness of the conjugate pad can be adjusted to adjust the incubation time of the sample and antibody for optimal results. Following the conjugate pad are the test and control lines. These consist of covalently bonded antibodies that bind to the target of interest (target line) or the labeled antibodies themselves (control line). When a sample is loaded in the lateral flow assay it first wets the sample pad followed by the conjugate pad. If the target of interest is present it will bind to the re-solubilized antibodies and the target-antibody complex will continue down the assay and bind to the antibodies in the target line. Additionally, some antibodies due to random chance, some antibodies will not bind to the target of interest or miss binding to the antibodies in the target line and bind to the antibodies in the control line. If no target is present in the sample the labeled antibodies will still re-solubilize and continue down the assay but will only be able to bind to the control line. The presence of the control line in the assay confirms that the reagents in the assay are active and were not denatured during storage, while the presence of the target line indicates that the target of interest is present in the sample. Lateral flow assays are the most widespread application of paper-based microfluidics due to their low cost, extended shelf life, and ease of use, however there are many other examples that extend the capabilities of paper-based microfluidic assays. Further information on many of these “advanced” paper-based microfluidic applications can be found in this excellent review article.
References
Grieshaber, Dorothee, Robert MacKenzie, Janos Vörös, and Erik Reimhult. "Electrochemical biosensors-sensor principles and architectures." Sensors 8, no. 3 (2008): 1400-1458.
Gong, Max M., and David Sinton. "Turning the page: advancing paper-based microfluidics for broad diagnostic application." Chemical reviews 117, no. 12 (2017): 8447-8480.
Parolo, Claudio, Amadeo Sena-Torralba, José Francisco Bergua, Enric Calucho, Celia Fuentes-Chust, Liming Hu, Lourdes Rivas et al. "Tutorial: design and fabrication of nanoparticle-based lateral-flow immunoassays." Nature protocols 15, no. 12 (2020): 3788-3816.
Wang, Rongsheng, Yin Zhang, Jianfeng Cai, Weibo Cai, and Ting Gao. "Aptamer-based fluorescent biosensors." Current medicinal chemistry 18, no. 27 (2011): 4175-4184.
Wang, Chao, Mei Liu, Zhifei Wang, Song Li, Yan Deng, and Nongyue He. "Point-of-care diagnostics for infectious diseases: From methods to devices." Nano Today 37 (2021): 101092.