
The Epigenetic Role of DNA Damage in G-Quadruplex Structures
DNA Repair - Part 1
Repairing DNA damage is an essential capability for humans and other multicellular organisms. Inability to repair DNA damage can lead to cells dividing randomly and the development of both benign and malignant tumors. The David Lab at UC Davis is working on understanding the molecular mechanisms of DNA repair as a first step in developing targeted therapies to prevent common cancers. In particular, the lab has expertise in understanding a variety of different DNA repair enzymes, and deficiencies in these enzymes can lead to colon cancer, Alzheimer’s disease, and metabolic syndromes, to name a few. In this blog, the first of a three-part series (see Part 2 and Part 3), we will explore the epigenetic role of DNA damage in G-quadruplex structures. Knowing more about this molecular mechanism will help researchers better understand how G-quadruplex structures can be exploited for gene regulation (Figure 1).
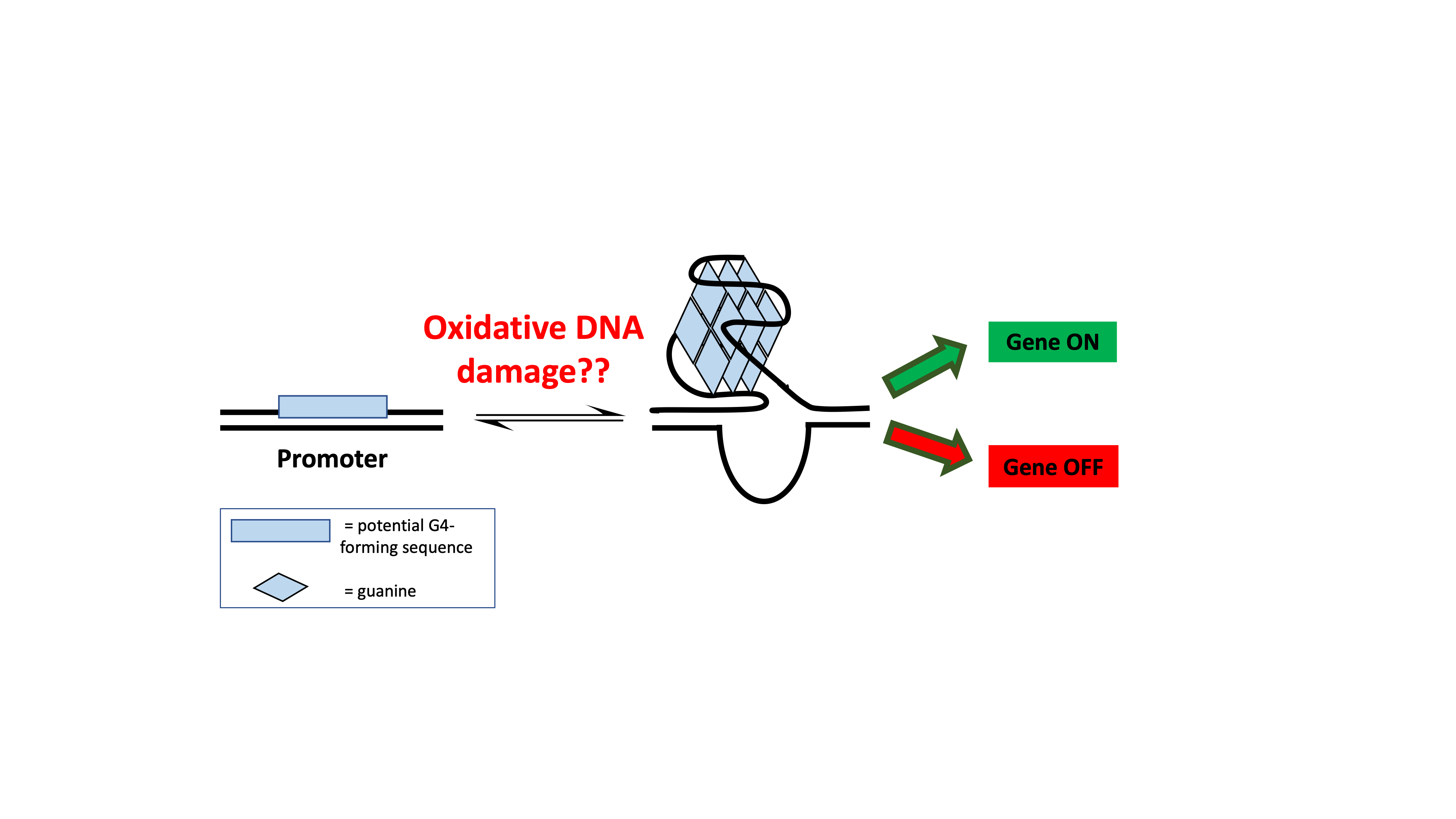
DNA damage can arise from a variety of different sources, such as ionizing radiation, pollution, and metabolism. Reactive oxygen species (ROS) are molecules that have the potential to damage all biomolecules in the body. In the case of DNA, the bases, sugar, and phosphate backbone are susceptible to oxidation by ROS. Guanine has the lowest reduction potential of the four standard bases (adenine, thymine, guanine, and cytosine), and is therefore the most likely to get oxidized. Oxidation of guanine can lead to the highly common oxidative product 8-oxo-7,8-dihydroguanine (OG), which introduces an oxo group at the 8-position of the base and a hydrogen at the N7 position (Figure 2).
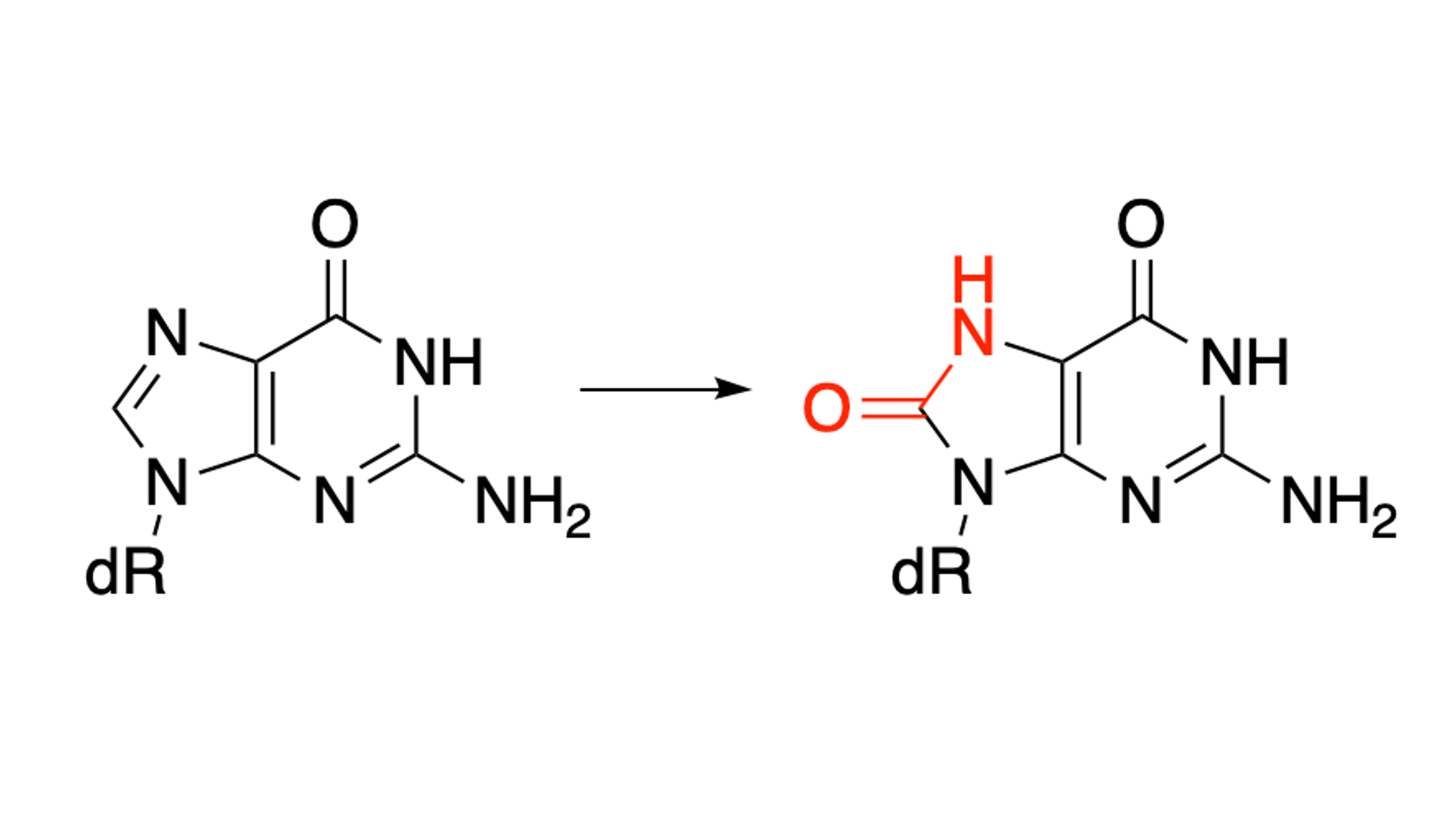
While guanine usually adopts the anti-conformation within DNA, as with all other base pairs, the 8-oxo group of OG causes the syn conformer to instead be favored. This causes the base to present a different hydrogen bonding face within the helix. Therefore, OG is particularly insidious because its syn conformation hydrogen bonding face mimics thymine, leading to stable OGsyn:Aanti mispairs. Upon a round of replication, OG can therefore be mispaired with adenine and a second round of replication can lead to G:C to T:A transversion mutations. The base excision repair enzyme hOGG1 is responsible for the repair of OG across from cytosine; however, if hOGG1 misses the repair of OG before replication occurs, MUTYH is responsible for the repair of OG:A mispairs before a transversion mutation is solidified. Following excision of the OG or adenine base, AP endonuclease I will continue the base excision repair process by processing the remaining apurinic/apyrimidinic site (AP site).1
While the most common form of DNA structure is the usual B form helix, DNA can exist in alternative structures throughout the cell. G-quadruplexes (G4s) are structures that form in single-stranded, guanine rich contexts. These sequences typically contain four or five “runs” of guanine residues (Figure 3). The guanine bases can Hoogsteen hydrogen bond into G-tetrads which then stack upon each other stabilized by central potassium ions located in the center of the structure. Because these structures are guanine rich, they are highly susceptible to oxidation by ROS.
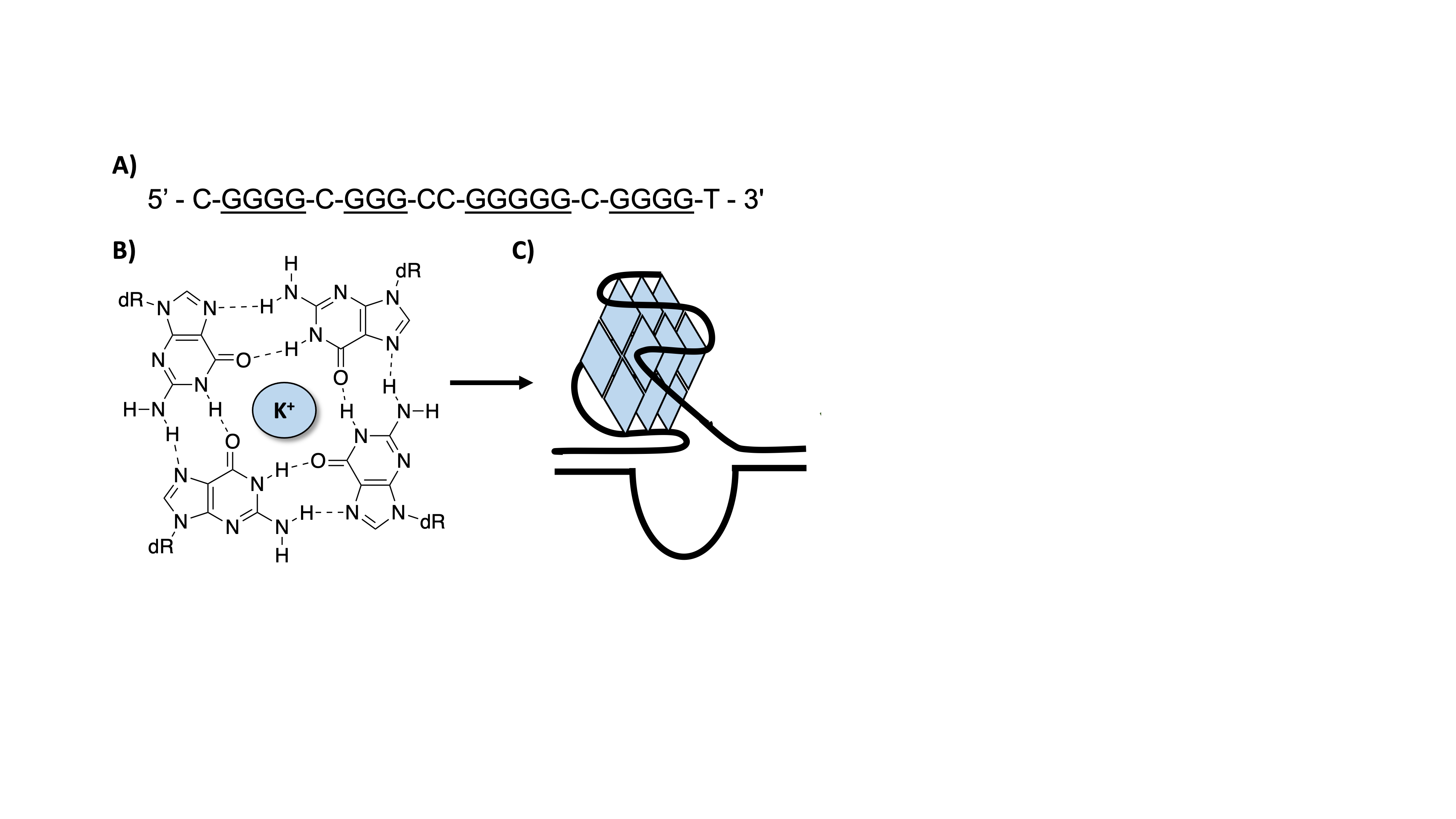
Potential G4-forming sequences (PQS) are found in significant prevalence in human gene promoters. The formation of these structures within the promoter regions has been demonstrated to affect subsequent gene expression. Therefore, G4s have become a recent hot topic for therapeutic targeting.2 It was previously unknown how the introduction of oxidative DNA damage into these G4 structures could potentially have impacts on gene expression.
The Burrows Laboratory at the University of Utah has developed a dual luciferase assay that allows for the monitoring of G4 structures as well as damage-containing G4s on gene expression (Figure 4).3 In this assay, a Renilla luciferase gene (Rluc) has a promoter region that contains the five guanine runs of the VEGF G-quadruplex sequence. This region also contains nicking endonuclease sequence sites that allow for the replacement of the G-rich sequence with a synthetic oligonucleotide containing a site-specific OG damage. The plasmid also contains a firefly luciferase gene (luc) that is used as an internal standard.
The authors incorporated OG into the plasmid’s G4 sequence in the promoter region at various positions. They then transfected the plasmid into human cell lines. Notably, the expression of Rluc increased the transcription of the reporter gene (Figure 4)!3 They then investigated if the repair of the OG affected transcription activation of the gene by transfecting the plasmid into WT and hOGG1 -/- cell lines and demonstrated that transcription of the gene is only increased in the WT cell lines. This indicated that repair of OG by hOGG1 was necessary to see an increase in gene expression. These results were in comparison to a sequence that was incorporated into the plasmid reporter that could not adopt a G4 fold, which did not enhance gene expression.
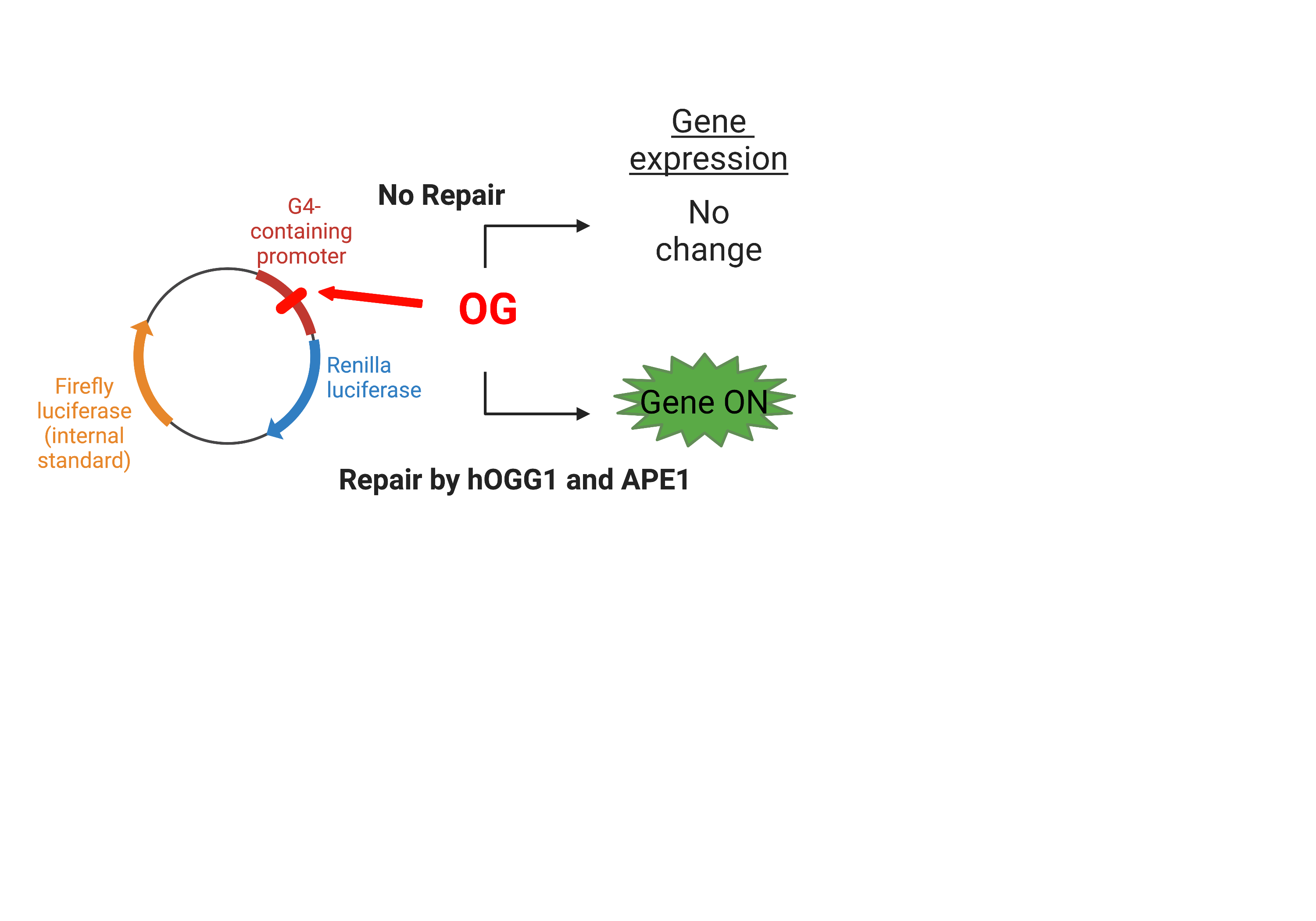
Because an AP site is formed after the OG is removed, the authors next examined if an AP site can also have similar effects on transcriptional activation. Using an AP site analog known as THF, the authors incorporated this into the plasmid reporter and again transfected the plasmid. Once again, they saw an increase in gene expression, and in the absence of APE1, gene expression once again diminished.3 Lastly, they examined if APE1 binding to the G4 sequence was required for gene expression by using an APE1 inhibitor that affects enzyme function but not DNA binding. Indeed, gene expression increased even in the presence of this inhibitor indicating that APE1 binding to the G4 was important for transcriptional activation.
Therefore, a mechanism of OG-induced gene activation within G4 structures has been proposed. First, base excision repair can be activated by the repair of OG by hOGG1 within a promoter region G4. The G4 structure can fold containing the AP site which APE1 will bind but poorly cleave, therefore increasing transcription. APE1 has been shown to interact with protein factors that bind promoter sequences to increase gene transcription, and the authors believe this mechanism is inducing gene expression.
The effect of OG within the promoter region G4 sequence has been demonstrated with other G4 sequences as well, including the KRAS and RAD17 G4s.4,5 Additionally, it has been shown that incorporating the OG into the template or the coding strand of the plasmid reporter can alternatively increase or decrease gene expression.6 The authors also investigated if an OG:A base pair was instead placed into the G4 sequence followed by repair by MUTYH, if gene expression is affected. They demonstrate that repair of A across from OG leading to the restoration of an OG:C base pair, followed by repair by hOGG1 of OG, gene expression changes could still be observed.6 Overall, these results demonstrate that OG can play an epigenetic role in the cell.
References
David, S. S.; O’Shea, V. L.; Kundu, S. Base-Excision Repair of Oxidative DNA Damage. Nature 2007, 447 (7147), 941–950. https://doi.org/10.1038/nature05978.
Ferino, A.; Xodo, L. E. Effect of DNA Glycosylases OGG1 and Neil1 on Oxidized G-Rich Motif in the KRAS Promoter. Int. J. Mol. Sci. 2021, 22 (3), 1–13. https://doi.org/10.3390/ijms22031137.
Fleming, A. M.; Ding, Y.; Burrows, C. J. Oxidative DNA Damage Is Epigenetic by Regulating Gene Transcription via Base Excision Repair. Proc. Natl. Acad. Sci. 2017, 114 (10), 2604–2609. https://doi.org/10.1073/pnas.1619809114.
Fleming, A. M.; Zhu, J.; Ding, Y.; Burrows, C. J. 8-Oxo-7,8-Dihydroguanine in the Context of a Gene Promoter G-Quadruplex Is an On-Off Switch for Transcription. ACS Chem. Biol. 2017, 12 (9), 2417–2426. https://doi.org/10.1021/acschembio.7b00636.
Tian, T.; Chen, Y.-Q.; Wang, S.-R.; Zhou, X. G-Quadruplex: A Regulator of Gene Expression and Its Chemical Targeting. Chem 2018, 4 (6), 1314–1344. https://doi.org/https://doi.org/10.1016/j.chempr.2018.02.014.
Zhu, J.; Fleming, A. M.; Burrows, C. J. The RAD17 Promoter Sequence Contains a Potential Tail-Dependent G-Quadruplex That Downregulates Gene Expression upon Oxidative Modification. ACS Chem. Biol. 2018, 13 (9), 2577–2584. https://doi.org/10.1021/acschembio.8b00522.